Biophotonics – The Science Behind Energy Healing, Part II
How Light Interacts With Biology
Biophotons and the work of Prof Fritz-Albert Popp
by Katrin Geist
Fritz-Albert Popp shared the fate of many an ingenious person: he got fired and ridiculed for a revolutionary rediscovery: cells contain and emit light. And what goes for cells also holds true for whole organisms, including humans. Everything living constantly emits an ultra-weak radiation. Popp called it biophoton emission, indicating its biological origin. To illustrate how weak this light is: it would equal still seeing a candle flame at a 20 km distance. This highlights a major challenge in science: it can only ever measure phenomena detectable by current equipment. Popp had long worked on developing a machine sensitive enough to detect single photon events. Beginning with cucumber seedlings, he measured low level light emissions (biophotons) coming from these plants when put into his photomultiplier machine (Fig. 6), a technology based on work by Albert Einstein who received the Nobel Prize for it in 1921. Popp noticed that any organism he tested emitted a weak light, and it is well established today that this pertains to all life forms (Bischof 1995).
What did this light emission mean? What could its function be? And could anything of such low intensity really matter to organisms?
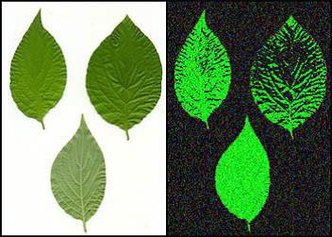
One clue came from his own previous work studying two forms of almost the same thing: 1,2 benzpyrene and 3,4 benzpyrene. While their structural difference is tiny, the former is harmless to us, and the latter is a highly carcinogenic substance (e.g. in cigarette smoke and coal-tar). When shining UV light on 3,4 benzpyrene, Popp noticed something baffling: the substance would not radiate back light at the same wavelength, but rather emit a different wavelength. Normally, and like the harmless 1,2 benzpyrene, things emit back the same frequency they were exposed to. This didn’t. Instead of radiating back the same UV wavelength, 3,4 benzpyrene emitted light of a different kind. It absorbed one light form and gave off another. It scrambled light frequencies. Popp was amazed to learn that 380nm, the wavelength at which his test molecules reacted as light scramblers, is part of the light spectrum at which DNA photorepair – a well known and accepted yet not fully understood mechanism – operates. One can next to destroy a cell with strong UV light or X-rays, for example, and then expose it to a weaker dose of UV light to induce cell repair. Cells recover almost completely within a few hours. This amazing ability is termed DNA photorepair or photoreactivation, and it depends on light exposure between 310nm and 400nm. Popp thought nature was too coordinated for this to be a coincidence – could it be that frequency scrambling substances were at the root of causing cancer? Had he even discovered the mechanism behind carcinogenic effects? What if light scrambling molecules interefere with and disrupt or prevent DNA photorepair, thus leading to mutations? He felt on to something.
Presenting his results at a conference, he was met with much skepticism and resistance. His results did not fit the mould and stretched attendees’ imagination too far. Despite this disappointing experience, Fritz-Albert Popp persisted with his inspiring research. Studying 37 more substances (some harmless, some carcinogenic), a pattern emerged: without exception, every frequency scrambling chemical turned out to be a carcinogen, absorbing at 380nm, and then emitting a different wavelength. Popp wondered about the implications of this for cells: the light absorbing property of carcinogens at 380nm with subsequent scrambling would block light of that wavelength, thus withholding it from photorepair, and eventually preventing it altogether. Consistent, long-term exposure (e.g. smoking) to carcinogens, then, would deprive cells of their repair mechanism and render them vulnerable to damage. It would also mean that, in order to carry out photorepair, cells naturally contain UV light (as it does not penetrate skin very deeply, and all cells use it), and that DNA interacts with it. Measuring his cucumber seedlings confirmed that organisms not only emit light, but coherent light at that (see below). Question remained: where did this inner light come from?
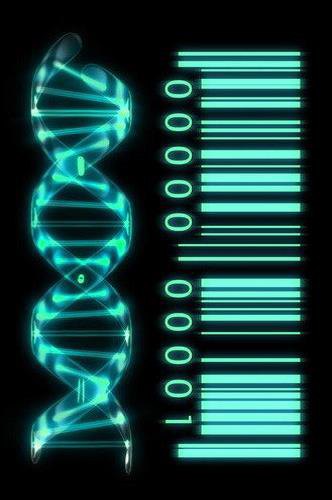
Popp mused it must come from the live food we eat. Green plants harness sunlight and convert it into biochemical energy. When we enjoy a fresh salad, we also ingest photons currently held within the plant tissue. There is good experimental evidence that DNA is a lot more than just a blueprint for building proteins. It seems to hold and emit photons (Rattemeyer et al 1981), allow for coherence (see below) (Popp 1984), and, recently, was used as digital information storing device, akin to a flash drive. Church et al (2012) stored (and retrieved) an entire book: 53.426 words, 11 jpg’s and one JavaScript program. Also, what has been termed “junk DNA” (some 95% of it), turns out to be anything but: remember the barcode? Figuratively speaking, it’s a temporary description of what’s going on in a cell, regardless of sequences coding for proteins (genes) or not (“junk”). Popp believes that DNA is a light storing resonator molecule that uses light signals to coordinate intra- and inter-cellular processes. (Resonators either generate waves of specific frequencies or select specific frequencies from an incoming signal. They temporarily store light.) DNA acts as an antenna sending and receiving light signals. Popp’s research, and that of others, certainly points in that direction (Popp 2006, Blank & Goodman 2011, Garo & Haener 2012, Meyl 2012).
Since every biochemical reaction requires energy for activation, photons make perfect sense to provide that, especially because they act like catalysts: without being used up in the process, they initiate a reaction, and then return to the light field, ready to repeat the task. In other words, a cell carrying out 100.000 reaction per second (!) does not require 100.000 photons to kick them off. A handful could do, since they are reused, and, as light, travel very fast. It’s similar to a honey bee visiting hundreds of flowers instead of only one. In a cell, everything is in constant flux and exchange, in a most coordinated way. And you consist of some 50 trillion cells. Imagine what’s going on at all times!
Also interesting in this respect is that every atom (basic unit of matter) functions as a resonator for a specific wavelength, and this translates up the scale to molecules (which consist of atoms), cells, tissues, and organs. What does this mean? Essentially, that everything is in motion in response to light waves which excite atoms at certain wavelengths. This excitation has atoms form molecules and molecules aggregate to larger structures like cell membranes. Photons provide the impetus for anything to happen. Everything vibrates. Why is this important? Every atom, molecule, cell, tissue, organ and person resonates with certain frequencies and not with others. That’s why we like some vibes or people, and not others. That’s what different tastes are, and different smells – different perceptions. When something resonates with us, we fell really good, in love even. We can resonate with people, places, foods or ideas. It generally just feels very good. It also means that every molecule and every cell serves as a potential light source, due to the nature of resonators as temporary light traps. This allows for the formation of three-dimensional light fields throughout the body. One could say that light glues the body together, as every cell emits and aborbs light in communication with its neighbours. Biophotons prefer spaces of constructive interference (amplified light fields) and interact with charged particles (e.g. electrons, atoms, proteins). Therefore, they influence the body’s charge distribution, and thus its energy field.
Coherent light as ordering principle
Coherent light (e.g. a laser beam) is light that originates from a very small source (e.g. a photon, or by passing through a pinhole, see Fig. 8). A word of many definitions, in the world of biophotons, coherence denotes 1) a high degree of order and 2) the ability to form light fields through wave interference (amplification & attenuation), where biophotons from spatially different sources interact to weave an ordered (coherent) light field. It is these standing waves that enable information exchange in biological systems. A standing wave keeps the same form over time. It is subject to boundary conditions (as given by a cell membrane or a river channel) which maintain and determine its shape. Change only one boundary condition, and the entire wave (system) changes: light fields are holistic. That is why coherence is so important, as it buys cells time to carry out tasks until coherence erodes, thus changing the quality of information exchange possible. For example, healthy people emit coherent biophotons with periodic patterns, whereas ill people show little coherence and disturbed or no periodicity (Cohen & Popp 1997). The ability of biological systems to achieve coherence exceeds that of modern lasers by orders of magnitude (Popp 2006).
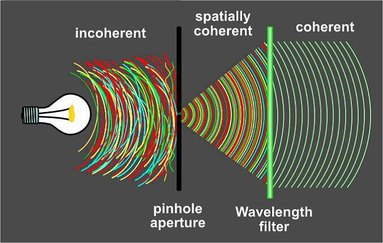
So by analogy, think of a packed rugby stadium. Photons are like people: they spontaneously and temporarily connect and cooperate as one to create order and meaning while keeping their individual identity. La ola, the wave, goes around the stadium with people participating in a cooperative pattern that carries meaning: that’s coherence. No single person could bring it about. But cooperative interaction can. Likewise, photons weave a tapestry of information in biological systems, thereby transmitting and communicating information about the state of the system. Forget the barcodes: think la ola! It’s more like a dance anyway.
Popp’s brilliant move was to calculate possible resonator wave patterns (light fields) for cells. The trouble with research of this kind is that one cannot directly measure cellular light fields – cells are too small for inserting probes directly, and that very act would also immediately alter the boundary conditions (by disturbing the cell membrane), and thus the result. So scientists resort to indirect approaches which involve measuring biophoton emissions to infer standing wave patterns (light fields) present in cells, and to theoretical calculations such as: what biophoton wave interference patterns (light fields) are possible within cellular boundary conditions? In cells, boundary conditions largely depend on the cell membrane: its shape, molecular composition and electrical charges, for example. A standing wave in a river may depend on channel width and depth, log jams and water flow. If only one boundary parameter changes, the resulting wave changes, too, whether that’s water or light. Fritz-Albert Popp’s genius and the foundation of his life’s work should surely see the Nobel Prize as far as I’m concerned. Amazing to think that this knowledge is… at least 35 years old. People all too often die over their great contributions without recognition. One can only hope that “they” make up their minds soon.
Posted in Other Topics, Science For The New Agewith comments disabled.